Transcatheter Aortic Heart Valve Replacment (TAVR) Research
Numerical and Benchtop Evaluations and Development of TAVR Valve Devices and Procedures
- Calcific aortic valve disease is a cardiovascular condition that causes the progressive narrowing of the aortic valve (AV) opening, due to the growth of bone-like deposits all over the aortic root (AR).
- Transcatheter aortic valve replacement (TAVR) is a minimally invasive procedure that has recently become the only lifesaving solution for patients that cannot tolerate the standard surgical valve replacement.
- This technique comprises of a prosthetic valve mounted on a stent and deployed inside the pathologic native valve.
Patient-specific In-Vitro Models for TAVR Performance Evaluation
Traditionally, TAVR valves are benchmarked and evaluated with the ISO 5840 standard,
which was originally designed for surgical valve testing, and negates key anatomical
features of aortic stenosis patients. The interaction between the TAVR stent frame
and the unique/ irregular calcific masses inside the patient leaflets define the deployed
shape and therefore the functionallity of the valve.
The goal of this research was to develop a patient-specific replica of calcific aortic
valve disease(CAVD) to emulate the expected clincial performance of the TAVR devices.
In collaboration with Vascular Simulations, we developed such a model to interface with the advanced simulation system called
the Replicator Pro, which allows us to study various patient anatomies inside the
cath-lab as if it was a patient on the table. We can rapidly deploy, measure and image
the valves for clinical evaluations as well as develop more adavanced imaging techniques
such as an intra-procedural CT for measure the coaption of the stent to the replica
lumen.
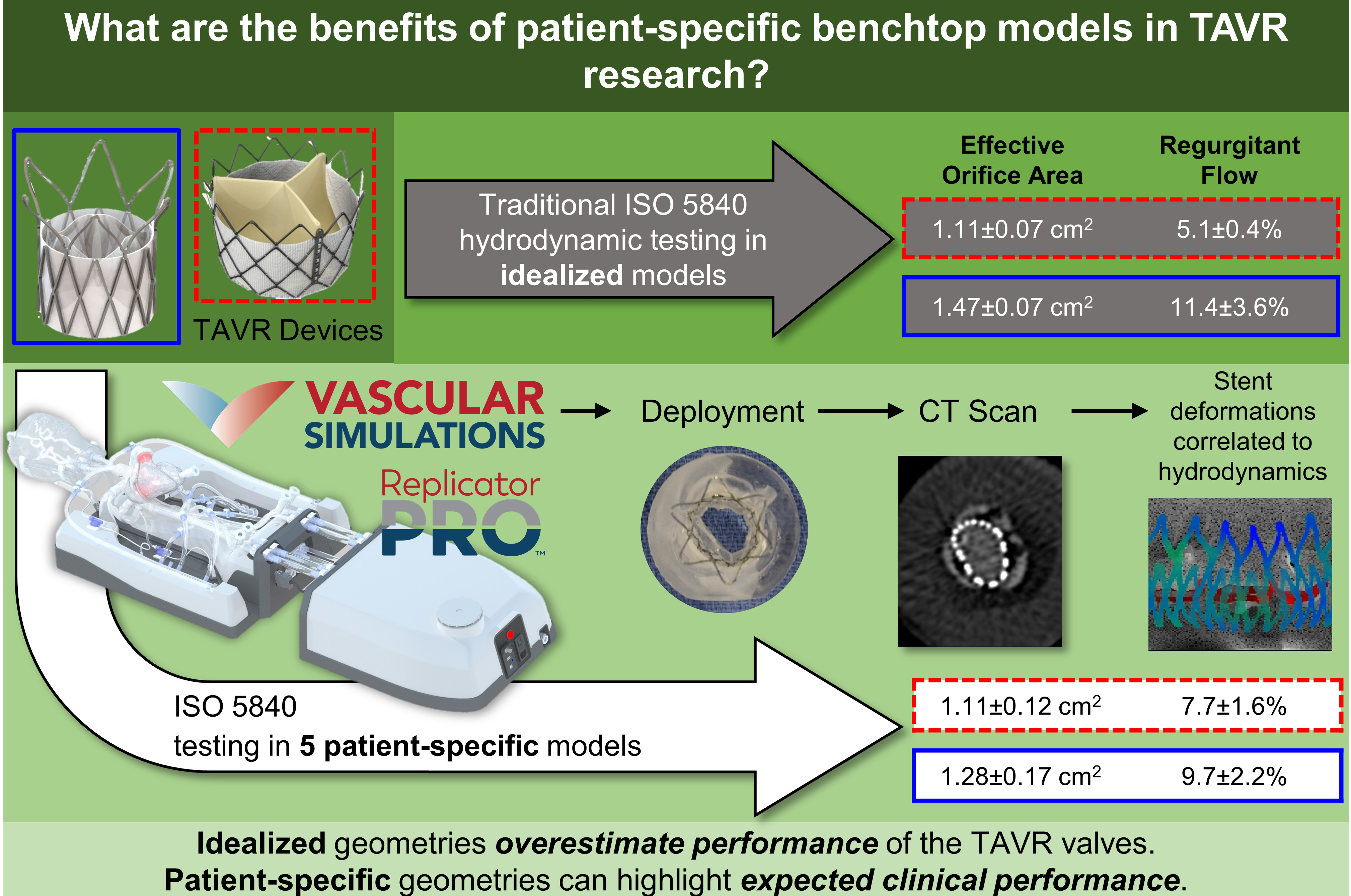
Two different TAVR devices, one self-expandable polymeric and one balloon-expandable tissue valve, were evaluated according to the ISO 5840 guidelines but in five different patient models. The patient-specfic results were compared to the idealized geometry simulator commonly used in ISO 5840 testing, and we showed that the current standards can dramitically overestimate the performance of the valves.
Evolving from in-vitro models to in-silico simulations
In order to study the resulting paravalvular leak flows in greater detail, we developed a protocol to µCT scan the deployed TAVR devices inside the CAVD replicas. Segmentation of the deployed geometry yeilds an ideal numerical domain to perform detailed CFD analysis (ANSYS Fluent) on the leak flows.
Lastly platelet-like particles are seeded with a DPM model in the ventricle geometry and tracked for 3 cycles. Showing the first evidence of the possiblity of platelet to enter the PVL domain during systole and the potential of repeated reincorporation into the PVL flows over the successive cycles.
- Expansion of TAVR makes fatigue life and durability crucial to device success
- Nitinol stents experience cyclic displacements/strains in vivo, yet benchtop stent frame fatigue testing excludes beating heart effects and dynamic loading
- In silico approaches include beating heart effects, more accurate modeling of TAVR device durability
- The electro-mechanical SIMULIA Living Heart Human Model of a beating heart allows for virtually deploying devices to analyze displacements, associated strains, tissue stresses and contact forces
- With this approach, we examined TAVR stent design implications on fatigue outcomes by scaling strut width by 20%
- The primary cause of element failure was strain amplitudes exceeding fatigue limits for nitinol, where the failure threshold was overlaid on fatigue plots to analyze stent elements for each model that had "failed" virtually
- Our PolyV-2 stent demonstrated superior fatigue resistance when compared to the comemrcial CoreValve and Evolut R stents models, as it was previously optimized to minimize stresses and strains
- Localized modes of stent failure highlighted here in current commercial devices demonstrates the utility of intricate in silico models to evaluate stent design for optimization of fatigue resistance
Mitigating Thrombogenic Risk By Varying Leaflet Design
- Current TAVR products are stented, bioprosthetic tissue valves whose durability and design is limited (structural damage during crimping)
- Novel polymers allow for more durable TAVR valves and mitigate current complications as they combine features of engineering flexibility, hemocompatibility, structural integrity and reproducibility
- TAVR is associated with long-term, life-threatening thrombotic events, a consequence of prolonged exposure of platelets to elevated, cumulative cyclic stresses; increased risk of platelet activation via stress accumulation
- Must develop alternatives to current tissue-based TAVR products as new devices must remain safe and functional for extended periods of time
-
Optimization of polymeric leaflets and valve design can improve hemodynamics and mitigate thrombosis; optimization via geometry changes can be achieved
- Virtual commercial TAVR models (26-mm) were generated to match the phsyical devices, while multiple polymeric valve designs (26-mm) were created by varying leaflet attachment and belly curvature
- With the constructed leaflets and stents, idealized TAVR deployments were generated and FSI analysis was performed for two cardiac cycles, where leaflet kinematics and valve hemodynamics were analyzed
- Validation of commercial devices via effective orifice area (EOA) and velocity profiles
- Probability density functions (PDFs) of the accumulated stresses for the flowing platelets were computed for each device (which represents the “thrombogenic footprint” for each respective device), analyze thrombogenic potential of different leaflet designs by comparing PDFs
- Here, we see the top 100 stress accumulation platelet trajectories colored by stress. In particular, we see the highest stresses near the surfaces of the prosthetic leaflets and at the leaflet tips, demonstrating the key idea that platelets interact differently with varying valve designs and experience different magnitudes of stress during their trajectories as they flow past each prosthetic device
- Hemodynamics and leaflet kinematics were different for each TAVR design, where designs #1-2 and the SAPIEN3 showed lower peak fluid velocities as compared to the other devices, likely due to these leaflets producing larger orifice areas and having reduced leaflet fluttering, which may be due to more optimal surface area and leaflet inflation
- The conversion of the data into PDFs also support this when looking at the tail region of the curves, as this region highlights the stress magnitudes that are likely to cause platelet activation. The original stress accumulation data was normalized to time in order to consider the small number of cardiac cycles simulated here. When doing this, the data distribution becomes unbiased and agrees well with the previous flow results since the commercial Evolut R, along with parametric designs #3-5 show the highest thrombogenic risk from the larger PDF tails
TAVR in BAV Patients - Thrombogenic Risk Analysis
- Current commercial TAVR devices often deploy elliptically in BAV patients, resulting in worse TAVR outcomes
- Our research group developed a polymeric TAVR leaflet design, PolyV-B, that was hypothesized to yield better hemodynamic performance (see TAVR Developement Page)
- We analyzed thrombogenic risk of this device and compared results to commercial devices currently used to treat BAV patients. Specifically, we modeled the 29-mm Evolut R (EV29) TAVR deployment first with the commercial leaflets in place, and then by replacing the commercial leaflets with our PolyV-B design, all in patient-specific models
- The novel design resulted in larger orifice areas and reduced jet velocities, outperforming the commercial Evolut R. The thrombogenicity comparison shows that the PolyV-B design has a smaller thrombogenic footprint when compared to the Evolut R
- In each panel, we see the red curve representing the commercial device was shifted towards higher stress accumulation values in addition to having more pronounced tail regions, increasing the likelihood of activating platelets. This is also indicated in the tabulated data, where the median stress accumulation among the highest or top 100 trajectories was largest for the Evolut R, indicating increased thrombogenic risk
Risk Assessment of Post-TAVR Cardiac Conduction Abnormalities Asing A 'Digital Twin' of Human Heart
- Post-TAVR Cardiac conduction abnormalities (CCAs) occur due to mechanical damage sustained by the atrioventricular conduction system located near the inter-leaflet triangle region between the non-coronary and the right-coronary leaflet. In his study, we have used an electro-mechanically coupled beating heart model to develop an in-silico technique to assess CCA risk incorporating the effect of a dynamic beating heart and pre-procedural parameters such as implantation depth and preexisting cardiac asynchrony in the development of post-TAVR CCA.
TAVR Deployment in Living Human Heart Model (Electro-mechanically coupled)
Preexisting conduction abnormalities modeling
- Contact pressure, Contact Pressure Index (CPI), and stress components on the area of interest (AOI) were analyzed for three implantation depths and three preexisting conduction abnormalities scenarios.
- Lower implantation, and preexisting-right bundle branch block (RBBB) poses higher risk of post-TAVR CCA which is aligned with the clinical findings.
Publication link- Assessing Post-TAVR Cardiac Conduction Abnormalities Risk Using a Digital Twin of a Beating Heart
Effect of Sinotubular Junction Size on TAVR Leaflet Thrombosis
- TAVR has emerged as a standard approach for treating severe aortic stenosis patients. However, it is associated with several clinical complications, including subclinical leaflet thrombosis characterized by Hypoattenuated Leaflet Thickening (HALT). A rigorous analysis of TAVR device thrombogenicity considering anatomical variations is essential for estimating this risk.
- In this study, we presented a comprehensive framework to analyze structural analysis of TAVR deployment, hemodynamic analysis via FSI simulation coupled with thrombogenic risk assessment via Lagrangian particle tracking.
Patient model reconstruction and TAVR deployment
Structural analysis of device eccentricity
Hemodynamic analysis
- A smaller STJ size impairs adequate expansion of the TAVR stent, which may lead to suboptimal hemodynamic performance.
- Larger STJ size marginally enhances the hemodynamic performance but increases the risk of TAVR leaflet thrombosis. Such analysis can aid pre-procedural planning and minimize the risk of TAVR leaflet thrombosis.
Publication link- Effect of Sinotubular Junction Size on TAVR Leaflet Thrombosis: A Fluid–Structure Interaction Analysis
Feasibility and Performance Analysis of Redo-TAVR
- Durability remains one of the major concerns for TAVR technology. This evidence of thrombosis and rapid degeneration of prosthetic valves poses a significant risk to the increasing number of young and low-risk TAVR recipients, necessitating repeat intervention. Transcatheter aortic valve-in-transcatheter aortic valve (TAV-in-TAV) or redo-TAVR is being considered as a potential treatment of choice for transcatheter heart valve (THV) degeneration which has shown success in addressing suboptimal outcomes.
- In this study, we leveraged advanced computational modeling to analyze structural and hemodynamic performance of 4 redo-TAVR combinations and suggested the pros and cons of easch combination.
Redo-TAVR Feasibility Analysis Framework